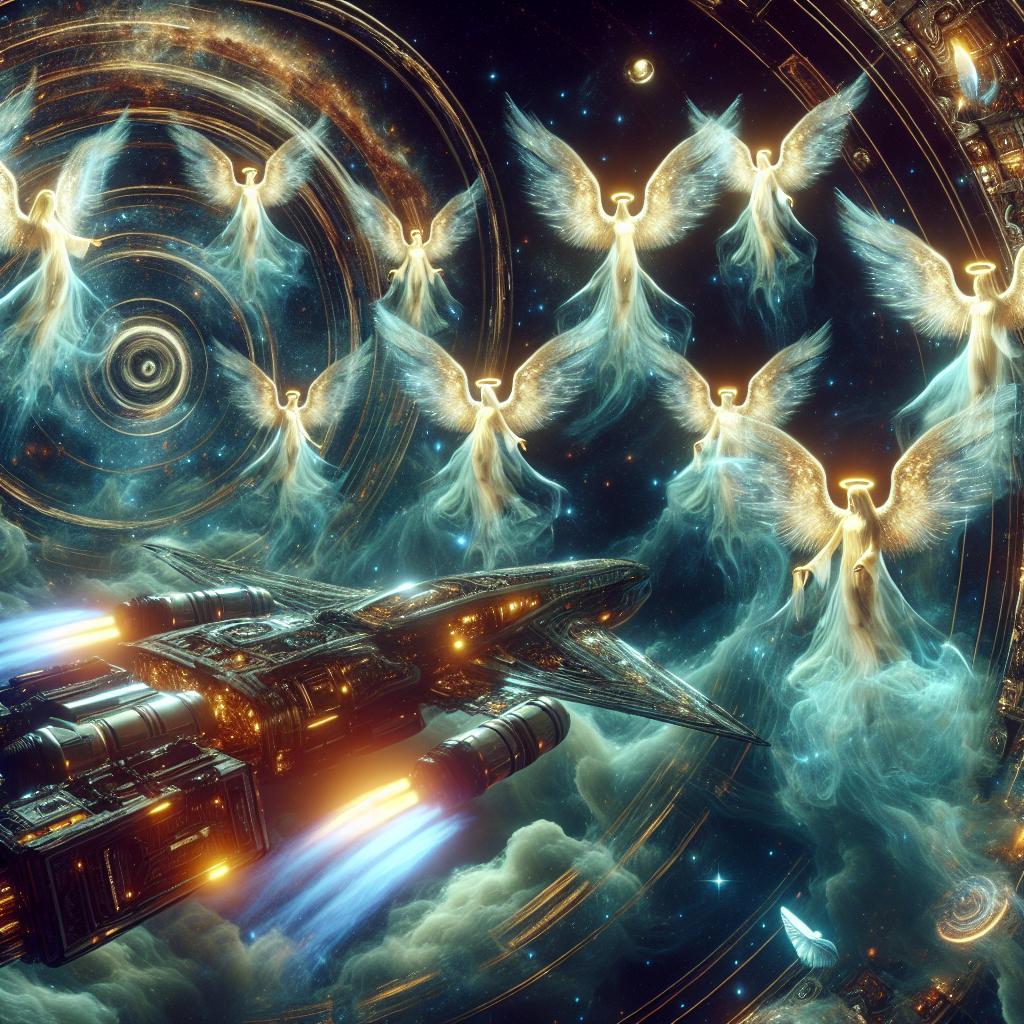
Overcoming G-Forces in Space Travel: A Christian's Guide to Resilience
Published: 08 April 2024
More Space Travel Problems: G-forces
Introduction
In the realm of space travel, there are numerous challenges that need to be overcome. One of these challenges is the impact of g-forces on astronauts. G-forces, which refer to the acceleration experienced by an object due to gravity, can have a significant impact on the human body. Understanding the effects of g-forces is crucial for ensuring the safety and well-being of astronauts during space missions.
What are G-forces?
G-forces are a measure of acceleration, calculated using Newton's Second Law, which states that force is equal to mass multiplied by acceleration (F = ma). On Earth's surface, the acceleration due to gravity is approximately 9.8 meters per second squared (m/s²), commonly rounded to 10 m/s² for simplicity. This means that objects experience an acceleration of 10 m/s² due to gravity.
Effects of G-forces on the Human Body
High g-forces can be detrimental to the health of astronauts, fighter pilots, and racing drivers. The duration and direction of these forces play a significant role in determining their impact. Short-duration exposure to high g-forces is generally better tolerated. For example, some Indy racing car drivers have experienced impacts in excess of 100 g without suffering serious injuries. However, even lower g-forces sustained for a longer duration can be fatal.
The direction of the g-forces also matters. "Downwards" forces, where blood rushes into the brain and eyes, can be particularly damaging. The limit for such forces in the downward direction is around -2 to -3 g (negative sign indicating downward direction). On the other hand, "forwards" or "eyeballs in" forces experienced during acceleration or when an astronaut lies on their back during rocket ascent are less dangerous. When decelerating, these forces are experienced in a backward-facing seat, which provides more protection during crashes. In general, g-forces perpendicular to the spine, known as "horizontal" g-forces, are the least dangerous.
G-Forces in Space Travel
Now, let's consider how g-forces come into play in the context of manned space travel. Assuming a speed of one-third the speed of light (c/3), which is still impractical for interstellar travel, we can explore the implications of sustained acceleration at extreme levels.
Research suggests that 25 g (approximately 250 m/s²) is likely beyond what the human body can withstand for more than a few seconds. Even assuming this extreme limit, it would take over 4.5 days to reach c/3 under such acceleration. This prolonged exposure to high g-forces could be extremely damaging if the spacecraft needed to stop suddenly to avoid a crash.
In fact, stopping a spacecraft traveling at c/3 would also require over 4.5 days of sustained deceleration at an equally damaging g-force. The stopping distance would be approximately 20 billion kilometers (12.5 billion miles), which is over twice the diameter of the outermost planet's orbit.
Turning in Space
Apart from accelerating and decelerating, space crafts also face challenges when changing direction. The acceleration experienced during a turn is at an angle to the direction of motion. To understand this concept further, let's consider the example of celestial bodies in orbit.
The moon orbits the Earth by constantly accelerating towards its center of mass. This acceleration is called centripetal acceleration and is directed towards the center. Similarly, the Earth constantly accelerates towards the center of mass of the Sun. When considering space crafts and their ability to turn, we need to examine how much acceleration they would undergo while changing direction at high speeds.
There is a simple formula to calculate the acceleration experienced by an object moving in a circular path:
a = v^2 / r
Here, v represents the speed of the object, and r is the radius of the circle. The velocity-squared term in this equation emphasizes the importance of speed. As speed increases, the g-forces experienced during a turn also increase significantly. Calculating the minimum turning radius given a maximum allowable acceleration of 25 g reveals that it would be around 40 billion kilometers (25 billion miles) or 267 AU (Astronomical Units).
To put this into perspective, a space craft attempting to turn as sharply as Earth's orbit would subject its passengers to approximately 6,800 g.
Why This Matters
Understanding the effects of g-forces on astronauts and space crafts is crucial for ensuring the safety and success of space missions. The limitations imposed by g-forces at high speeds make interstellar travel at a significant fraction of the speed of light impractical, if not impossible, using current technology.
Think About It
The challenges posed by g-forces in space travel highlight the remarkable design and precision required for human exploration beyond our planet. These limitations prompt us to consider the unique conditions necessary for life on Earth and the extraordinary care with which our Creator has fashioned our bodies to thrive within these conditions. Exploring outer space not only deepens our understanding of the universe but also illuminates the intricate balance and purpose inherent in God's creation.