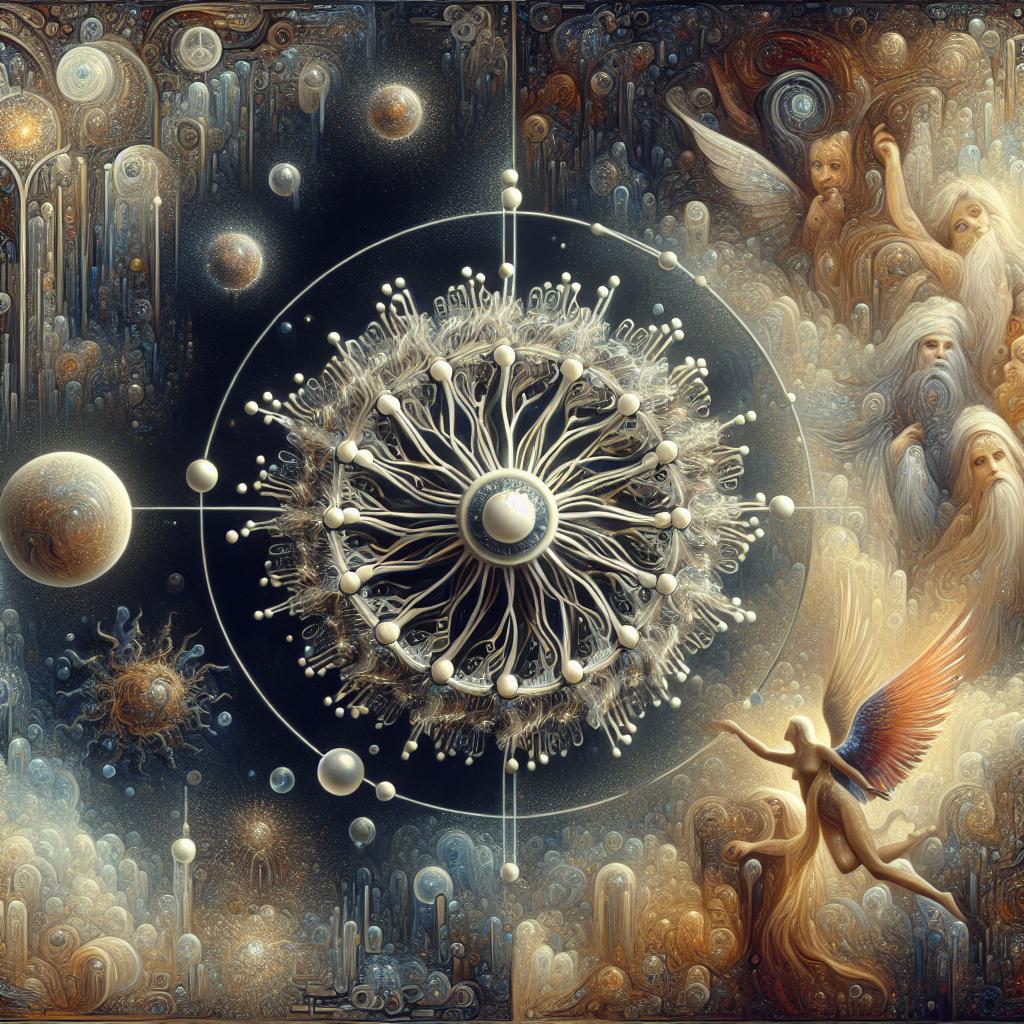
Unlocking God's Healing Power: The E. coli Motor Mystery Revealed
Published: 15 April 2024
E. coli's Electric Motor: A Marvel of Design
Did you know that the tiny bacterium E. coli possesses an extraordinary electric motor that allows it to swim through our gut? This remarkable motor spins a whip-like flagellum at an astonishing speed of up to 22,800 revolutions per minute! Some bacteria can even reach an incredible 102,000 rpm. The flagellum acts as a propeller, propelling E. coli through its environment.
E. coli's motor is incredibly small, measuring only about 45 nanometers in width. To put this into perspective, you could line up 2,000 of these motors across the width of a human hair! Scientists have marveled at this "remarkable nanomachine" and described it as a "sophisticated rotary motor" and an "example of elegance in molecular engineering."
Motor Parts
The motor of E. coli is composed of various parts that perform similar functions to those found in human-designed motors. These parts include gears, a rotor, axles, a driveshaft, bushings, a universal joint, and adaptor rings. Just like our motors, the bacterial flagellum is powered by electricity. The cell membrane of E. coli acts as an efficient capacitor that maintains an electrical difference by keeping positive and negative charges apart. Proton pumps within the cell remove hydrogen ions (protons) and create a positive charge outside the cell. These protons then flow back into the cell, creating an electric current. As these protons flow through the flagellar motor, they cause it to spin.
At the base of the motor is a central gear surrounded by up to 11 powered gears (or even 18 in some species). The flow of protons through these gears causes them to rotate, thereby driving the central gear. The powered gears rotate around axles anchored to the cell wall.
The rotor serves as a connection between the central gear and the driveshaft. It consists of an inner ring, an outer ring, an adaptor ring, and a socket that houses the driveshaft. The driveshaft passes outside the cell through a ring of proteins that function as bushings. These bushings are designed to minimize friction and allow the driveshaft to rotate with almost no resistance, making the motor highly efficient.
The motor also features a hook-shaped universal joint with specialized proteins that smoothly expand and contract as it rotates. This design makes the joint resistant to twisting while remaining flexible enough to bend, which is crucial for efficient movement.
Gear Mechanisms
E. coli's motor incorporates two gear-shifting mechanisms: one to change the direction of rotation and another to adjust power output. The motor can switch between forward and reverse gear in less than a millisecond by altering the diameter of the central gear's top section. This rapid gear-shifting enables the bacterium to navigate its environment effectively.
The second gear mechanism functions similarly to gears on a bicycle. When E. coli encounters thicker fluid, making it more challenging to rotate its propellers, torque sensors within the motor detect this change. The motor responds by engaging more powered gears with the central gear, increasing the rotational force produced by the motor. Conversely, if E. coli swims into thinner fluid, it disengages some of the powered gears to conserve energy. This sophisticated gear mechanism ensures that the motor operates at optimal efficiency by using only the necessary power.
E. coli's Navigation System
While E. coli's electric motor is impressive on its own, it would be useless without a navigation system to steer the cell. The bacterium navigates its surroundings using a highly precise system that tracks chemical gradients. This system allows E. coli to turn by switching the rotation direction of its 5-10 flagella.
The navigation system features a beautifully organized hexagonal architecture that computes thousands of binary input signals from various sensors at the front of the device. These sensors detect different chemicals, enabling the bacterium to respond to its environment. The system includes a short-term memory feedback loop, which allows it to compare chemical concentrations over time. Different sensor types communicate with one another to amplify signals up to 50-fold. This sophisticated signaling network provides the navigation system with high sensitivity, a wide dynamic range, and impressive signal amplification.
It's worth noting that E. coli's navigation system is relatively simple compared to those found in other bacteria, many of which are far more complex. This complexity highlights the intricate design and engineering present in bacterial systems.
Motor Construction
The construction of E. coli's electric motor is a complex process that involves numerous machines, motors, and proteins working together. The level of complexity involved in constructing the motor far surpasses the complexity of the motor itself. Let's explore the key steps involved in motor construction:
-
Producing Chemical Fuel: E. coli must produce a chemical fuel called ATP to power many of the assembly machines involved in motor construction.
-
Transporting Proteins: Newly manufactured proteins must be transported to an export machine while being protected during their journey.
-
Creating an Opening: E. coli must cut a hole in its cell wall for the driveshaft to pass through without damaging or bursting the cell.
-
Temporary Scaffold: To assist in the assembly of other proteins, E. coli forms a temporary scaffold structure.
These tasks require collaboration among multiple machines along complicated production lines within the bacterium. Some machines have proofreading systems that ensure tasks are performed correctly, while others can call on repair machines to fix any mistakes that occur during construction.
The proteins involved in motor construction come with identification tags that help identify, sort, and export them. The export machine controls the timing of protein delivery and communicates with machines at the start of the protein production line to prevent any backlog. Additionally, the export machine possesses a built-in rotary motor that unfolds the proteins, while other machines at the assembly site refold them into their proper positions.
Motor construction occurs in three stages. In the first stage, a genetic program processes input signals from various sensors within the cell and decides whether the conditions are suitable for flagellum production. The core structures of the motor and the hook are then assembled. Next, the propeller filament, navigation system, and powered gears are constructed. The final step of this stage involves building a precisely sized hook that measures 55 nanometers in length. During this process, a molecular ruler is used to measure the length of the hook to ensure optimal functionality.
The timing and logistics of the construction process are overseen by a sophisticated genetic program that functions similarly to a computer program. This program consists of over 50 genes organized into groups called operons, which are further categorized into three classes. These genes contain instructions for producing specific proteins that can switch other genes on or off within the program, forming a complex genetic circuitry. The production of different proteins is prioritized based on when they are needed at the assembly site.
The Impossibility of Evolution
The complexity and intricacy of E. coli's electric motor raise significant questions regarding its evolution through random mutation and natural selection. Evolutionists have previously suggested that the flagellar motor evolved from an injectisome, a needle-like apparatus used to inject proteins into other cells. However, recent research comparing the atomic-level structures of these two systems revealed significant differences, making it considerably more challenging for one to evolve into the other.
The flagellar motor's construction involves numerous interdependent parts that must all be present and functional for the motor to work properly. This poses a challenge to gradual, Darwinian evolution, which would need to invent all necessary parts simultaneously rather than through incremental steps.
Why This Matters
Understanding the complexity and design of E. coli's electric motor challenges the mainstream evolutionary explanation for its origin. The precision and sophistication of this motor suggest the involvement of an intelligent designer rather than a purely naturalistic process. This realization has significant theological implications and highlights the glory of our Creator, Jesus Christ.
Think About It
Consider the intricate design and engineering present in E. coli's electric motor. Reflecting on this complexity, what does it reveal about the nature of our Creator? How does this understanding deepen your appreciation for His handiwork?